Alexander Walter
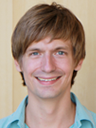
Speaker of Workshop 3
Will talk about: A catalytic slot model for exocytosis with a single release sensor effectively explains Ca2+-dependent properties of neurosecretion
Alexander Walter’s work focusses on presynaptic reactions mediating neurotransmitter release, with emphasis on proteins controlling the timing of exocytosis. Alexander obtained his PhD in the department of Erwin Neher at the Max-Planck Institute of Biophysical Chemistry, where he investigated the role of the neuronal SNARE proteins and the calcium-sensor synaptotagmin-1 for exocytosis by combining mutagenesis with patch-clamping. In addition to electrophysiology and molecular perturbation studies, Alexander is interested in combining these approaches with kinetic modelling of exocytosis. With this synergistic approach, he aims to scrutinize the function of individual proteins in specific reactions of the exocytosis cascade.
Communication between nerve cells in the brain relies on chemical transmission of signals across synapses, highly specialized cell-to-cell interfaces. At the presynapse, regulated secretion of neurotransmitter-containing vesicles, exocytosis, activates postsynaptic responses. Exocytosis occurs on different timescales: fast (synchronous) release is mediated by high-release probability vesicles, whereas vesicles with low release probability contribute to slow (asynchronous) release. However, to what extent those vesicles differ in molecular composition, placement or state of maturation remains controversial.
While previous models of exocytosis necessitated parallel calcium-sensors to account for the observed remaining slow exocytosis following deletion of the fast calcium-sensor Synaptotagmin, we found that a simpler, sequential model with only a single release sensor suffices to describe previously obtained data in chromaffin cells and neurons: we suggest that during maturation vesicles associate with a catalyst at the release site. This catalyst facilitates priming by a calcium-dependent increase of the interconversion rate between un-primed and primed vesicles without changing the population of those states in equilibrium.
Previous kinetic models describing exocytosis have mainly relied on the numerical integration of chemical kinetic equations, disregarding the quantal nature of vesicle transitions. Given the relatively low number of vesicles contributing to exocytosis at single synapses, this is problematic. To describe the natural condition more accurately, we developed a new approach to model exocytosis from neurons by combining a stochastic simulation algorithm, stochastic placement of release sites and realistic spatio-temporal modeling of calcium-signaling.
Our model can explain salient observations, including calcium-dependence of exocytosis components, their fusion and recovery kinetics as well as statistical features of synapses and their short-term plasticity. It also accounts for a number of observations not easily explained in earlier models, including submaximal release (decreased fraction of fast/slow release at low calcium concentrations), effects of regional SNARE-mutation and the phenotype of synaptotagmin knockouts when stimulated by high-frequency trains.
We provide a mathematical framework that can be used to investigate the impact of changes in calcium-channel-release site topology and suggest that calcium-dependent catalysis is a fundamental feature of the release apparatus, optimizing the refilling of fast vesicles during sustained stimulation.