Margarita Behrens
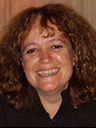
Keynote lecture
Will talk about: The epigenome and brain circuit changes during postnatal development
Margarita Behrens studied Biochemistry at University of Chile and received her PhD in Biochemistry and Molecular Biology from the Autonoma University in Madrid, Spain in 1990. After postdoctoral training in Molecular Biology at the Biomedical Institute in Madrid, in 1994 she moved to Neurology department at Washington University School of Medicine in St. Louis, Missouri. Working in the Center for the Study of Nervous System Injury, first as an Instructor in Neurology and later as a Research Assistant Professor, she studied signaling mechanisms involved in neuronal death. In the year 2000, she moved to the San Diego area and turned her attention to the basic neurobiology of neuropsychiatric disorders, specifically schizophrenia, focusing on the role that maturation of cortical inhibitory systems may play in the origin of the disease. She is, at present, a Staff Scientist at the Salk Institute for Biological studies and directs the experimental laboratory within the Computational Neurobiology Laboratory directed by Terry Sejnowski. Her laboratory studies the molecular mechanisms of the pro-psychotic effects of NMDA receptor antagonists in rodents as a validated model of schizophrenia. Work in her laboratory showed that repetitive exposure to the NMDA receptor antagonist ketamine leads to the loss of the GABAergic phenotype of parvalbumin-positive fast-spiking (PV) interneurons, a neurochemical hallmark of the schizophrenic brain. She discovered the role of oxidative stress, specifically production of superoxide radicals, as the initial event after NMDA-R antagonist exposures, leading to the loss of GABAergic markers and dysfunction of the fast-spiking parvalbumin positive subset of interneurons in cortex. Her current studies are focused on the determination of critical periods of vulnerability to oxidative stress during brain postnatal development, which when altered produce lasting perturbations at the neuronal and circuit level. She uses in vitro and in vivo approaches to characterize the molecular mechanisms underlying the heightened sensitivity of parvalbumin-positive fast-spiking interneurons in animal models of schizophrenia. Her projects encompass genome-wide transcriptome and methylome analyses, basic neurochemistry/neurobiology and physiology of fast-spiking interneurons to analyses of behavior and network activity by electroencephalography in awake animals.
During the period between birth and adulthood the brain undergoes profound changes driven by experience-dependent plasticity and the formation of stable neuronal circuits that last for the life-time of the individual. These changes are driven by changes in transcriptional patterns in each brain cell-type without the creation of new cells, suggesting the possibility that dynamic changes in DNA-methylation (mC) patterns could be driving these transcriptional changes. DNA methylation is a stable covalent modification that persists in post-mitotic cells throughout their lifetime, defining their cellular identity. However, the methylation status at each of the ~1 billion cytosines in the genome is potentially an information-rich and flexible substrate for epigenetic modification that can be altered by cellular activity.
Addressing this question requires integration of large-scale DNA methylation data sets with computational analyses capable of identifying dynamic epigenetic patterns throughout the genome. To do this, we produce whole-genome bisulfite sequencing data, at single-base resolution, from human and mouse frontal cortex throughout their lifespan. Using statistical and machine learning approaches such as high-dimensional cluster analysis, we found widespread methylome reconfiguration during fetal to young adult development, coincident with synaptogenesis. During this period, we found that highly conserved non-CG methylation (mCH) accumulates in neurons, but not glia, to become the dominant form of methylation in the human neuronal genome. We also found an interesting mCH signature that identifies genes escaping X-chromosome inactivation. Finally, whole-genome single-base resolution 5-hydroxymethylcytosine (hmC) maps revealed that hmC marks fetal brain cell genomes at putative regulatory regions that are CG-demethylated and activated in the adult brain.